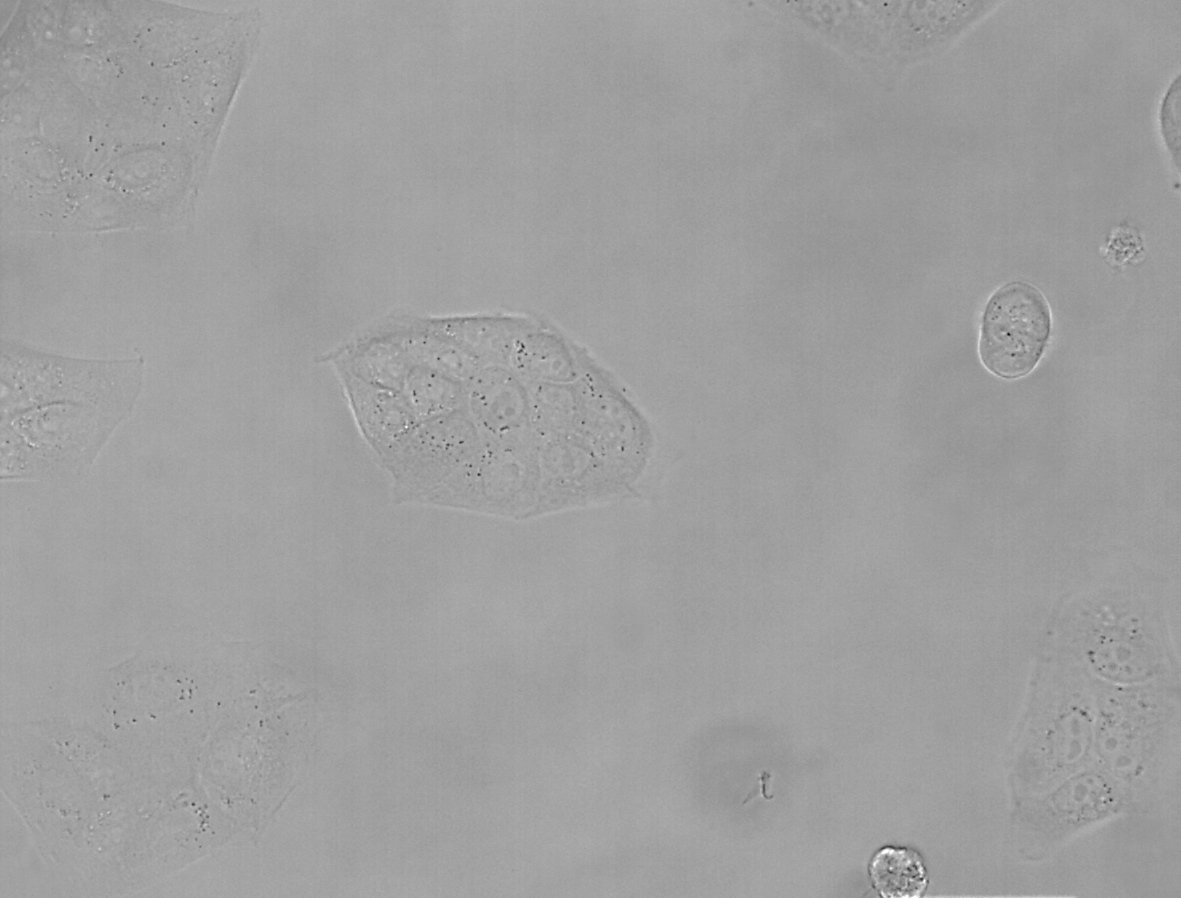
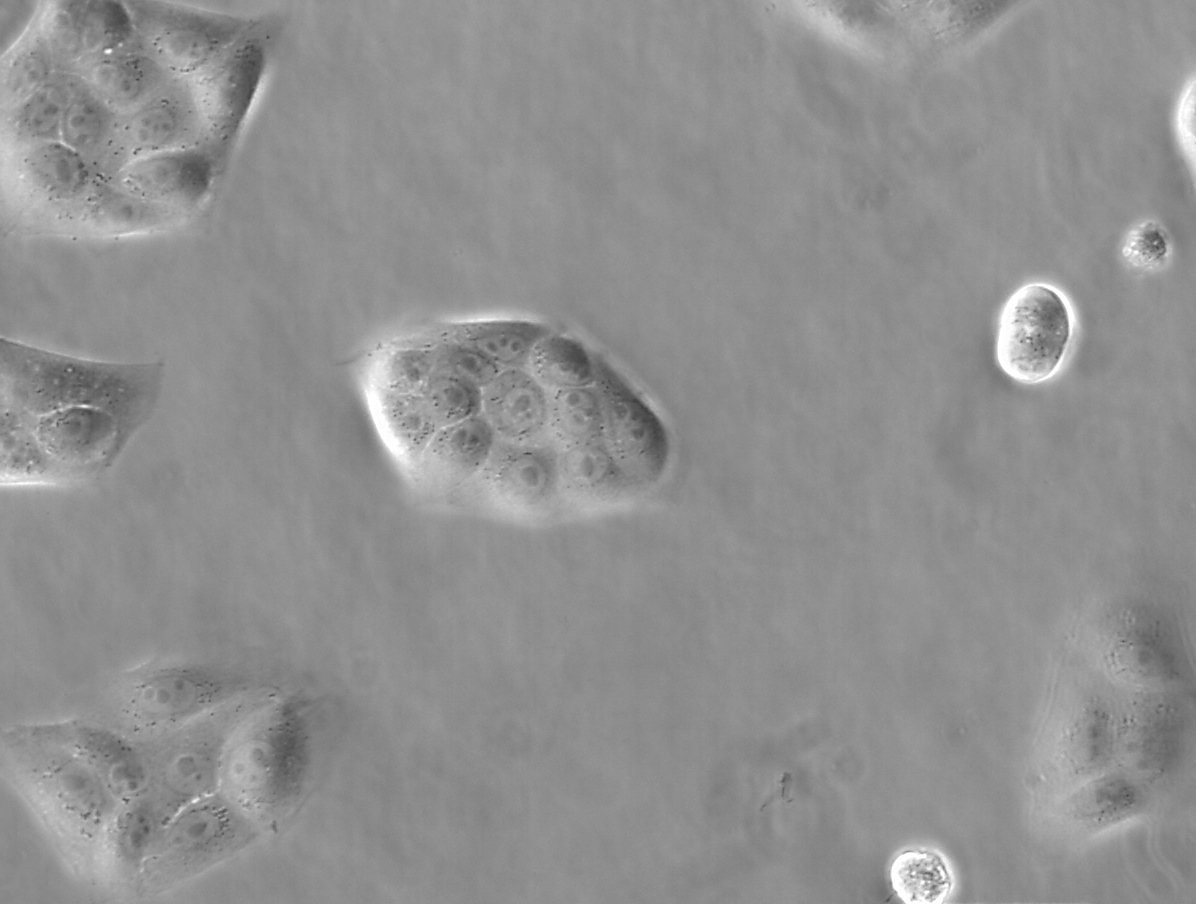
Figure 1: Images of MDCK (Madin-Darby canine kidney) cells taken with phase contrast and brightfield.
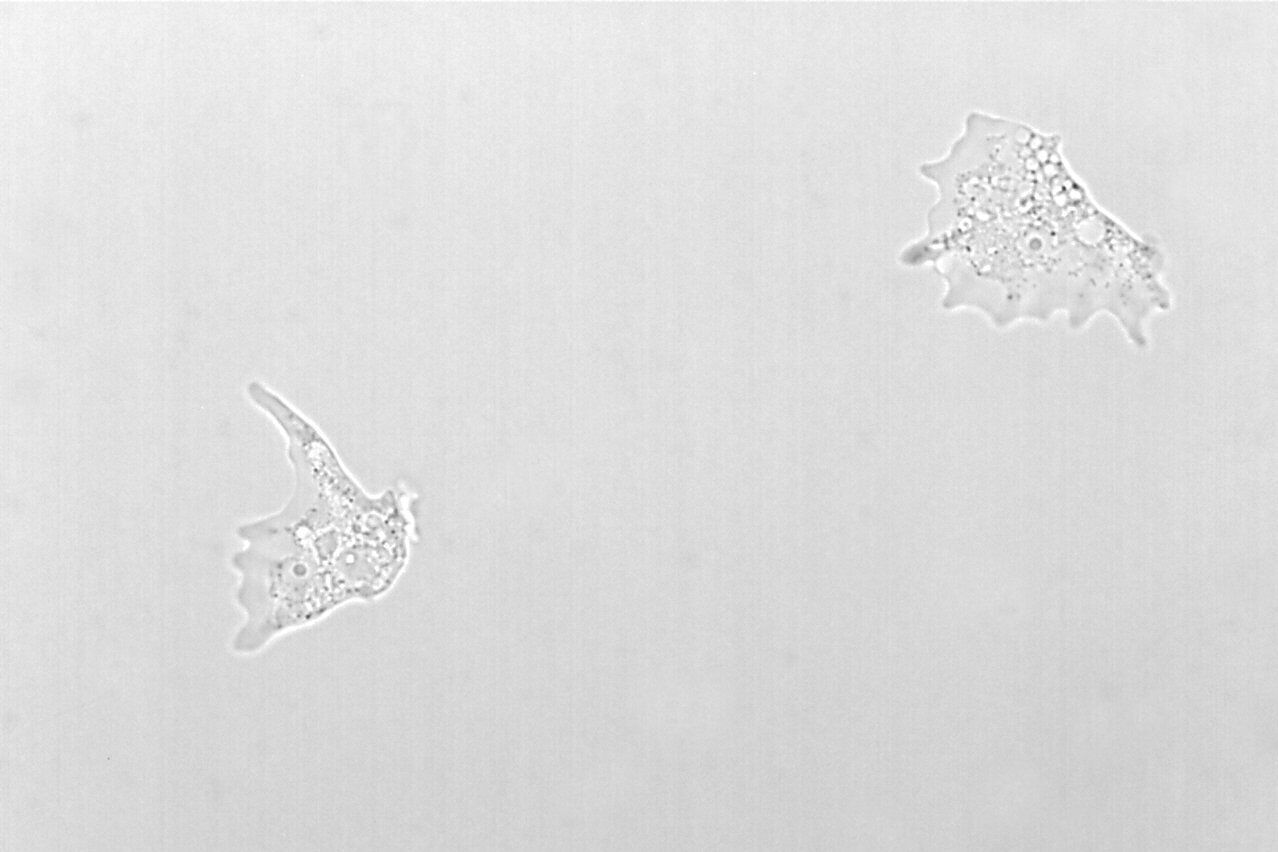
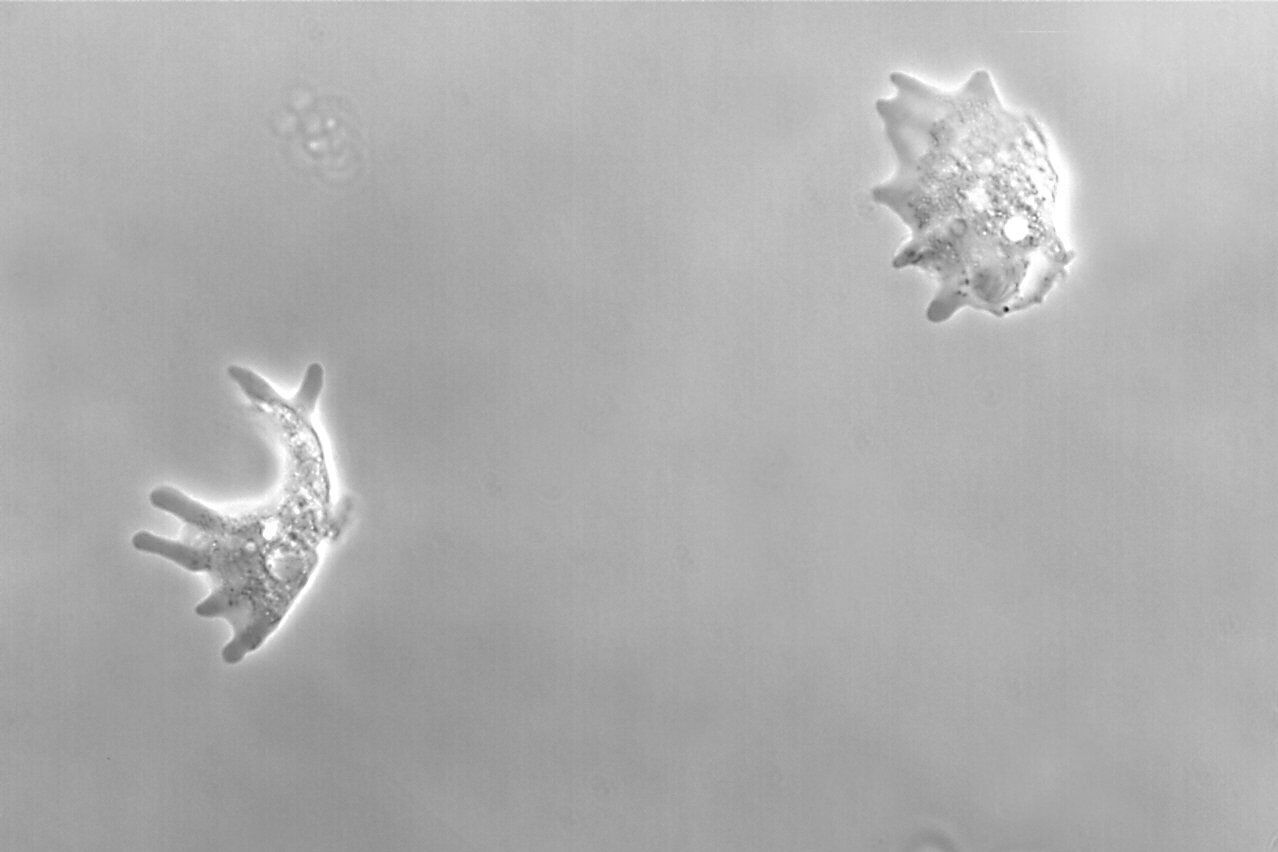
Figure 2: Images of Amoeba proteus, a unicellular organism, taken with phase contrast and brightfield.
The interference of light wave
The optical path length (OPL) is the product of the refractive index, n, of a medium and the distance, d, a light ray travels between two points in a medium: OPL = nd. An optical path may require the light ray to transverse more than a single medium, such as a gas, liquid, or solid. Common examples include light passing from air to water or air to glass. The optical path length is related to the transit time and velocity of light. The differences in the optical path length lead to different velocities of the light waves when they pass through a specimen, i.e., a phase shift. As a result, differences in phase occur. A higher refractive index, compared to the surrounding medium, leads to a deceleration of the light wave and a decrease of its phase.
Interference describes the interaction of two waves with each other and the resulting formation of a new wave pattern following the principle of superposition. The relevant parameter for the interference of light waves is their amplitude. If two waves interfere, the amplitude of the resulting light wave will be equal to the vector sum of the amplitudes of each interfering wave.
If the amplitude of the resulting wave is increased, the interference will be described as constructive. This will be the case if either two wave crests or two wave troughs meet at the same point in time. It is also possible that a crest of one wave and a trough of another wave meet at the same point in time. This case leads to a resulting wave with decreased amplitude. The interference between these two waves is then called destructive.
Optical path in a phase contrast microscope
The key elements of a phase contrast microscope are an annular aperture and a phase plate. The aperture in the form of an annulus is placed in the front focal plane of the condenser lens and limits the angle of the penetrating light waves. The phase plate lies in the back focal plane of the objective lens and has a phase ring made of a material that dims the light passing through it and changes its phase by λ/4, where λ represents the light’s wavelength.
In phase contrast microscopy under the conditions of Köhler illumination [5], the light waves which are not interacting with the specimen are focused as a bright ring in the back focal plane of the objective. The light ring spatially matches the phase ring along the optical axis and causes a phase shift of the undeviated light. Light that is diffracted by the specimen does not predominantly strike the phase ring and, therefore, is not affected.
Between the affected and the unaffected light waves there is a total phase shift of up to λ/2. The phase of undeviated light is advanced by λ/4 at the phase ring and light waves diffracted or scattered when passing through the biological specimen are usually retarded by λ/4. The total phase shift of λ/2 allows destructive interference of the light waves in the image plane. To enhance contrast, the undeviated light is dimmed when passing the phase ring which also absorbs some of it. It is important to avoid that the undeviated light is much brighter compared to the deviated light or the contrast will be poor.
A phase shift of λ/2, as observed in phase-contrast microscopy, gives rise to a maximal destructive interference effect as the crest and trough of light waves effectively meet at the same point in time. Therefore, the amplitude of the resulting light wave is reduced and the phase shift of the phase object is transformed into an amplitude shift.
Positive and negative – two forms of phase contrast
There are two forms of phase contrast: positive and negative phase contrast. They mainly differ by the phase plates used. For positive phase contrast, the phase of light passing through the phase ring is increased compared to the deviated light, whereas its phase is decreased for negative phase contrast. The retardation of phase in negative phase contrast leads to a destruction of the waves due to the phase differences. The light waves are in phase and, instead of destructive interference, constructive interference occurs which leads to an increased amplitude in the resulting light wave.
In positive phase-contrast microscopy, objects with a higher refractive index than the surrounding medium are displayed darker than objects with a lower refractive index. For negative phase contrast, the opposite applies.
Interpreting the phase contrast image
Phase contrast microscopy visualizes differences in the optical path length of light which passes through a specimen. The optical path length is related to the specimen’s thickness and refractive index. Cellular structures like plasma membranes and organelles have a profound impact on the optical path length. As many cells (especially in cell cultures) have a flat and regular shape, they are hardly visible with brightfield microscopy.
A phase contrast image of such cells amplifies differences in cell structure and can be regarded as an optical density map, because optical density has a great influence on the refractive index of a specimen or sample. However, several effects complicate the correct interpretation of the phase contrast image, as they do not directly rely on differences in optical path length.
The halo effect describes the appearance of a bright edge for positive phase contrast or a dark edge for negative phase contrast around large phase objects. Halos form because some of the diffracted light from the specimen traverses the phase ring as well. The ring of light formed by the undeviated waves is a little bit smaller than the phase ring and low-spatial-frequency diffracted light waves from the specimen can pass through the annulus. The deviated light passing through the phase ring maintains a phase difference of 90° and is therefore not affected by destructive interference. This leads to a reversion in contrast and causes the halo appearing at the boundaries of large objects.
The shade-off effect describes a situation where homogenous parts of a specimen are displayed with the same light intensity as the surrounding medium. Although the light passing through these regions experiences a phase shift, only minor diffraction occurs and the angle of scattering is greatly reduced. Therefore, these light waves enter the phase ring, like the undeviated light, and do not experience interference.
Another problem in phase contrast microscopy can be contrast inversion. If there are objects with a very high refractive index next to objects with a low refractive index, they will appear brighter instead of darker (for positive phase contrast). In such regions, the phase shift is not the usual shift of λ/4 for biological specimens and, instead of destructive interference, constructive interference occurs (it is the opposite for negative phase contrast).
Although these effects can render interpretation of phase contrast images difficult, phase-contrast microscopy is a convenient and important optical contrast technique for imaging phase objects. Additionally, phase contrast microscopy enables the investigation of cellular functions and structures in live specimens, making it the most frequently used contrast method for biological research.