Rapid Semiconductor Inspection with Microscope Contrast Methods
Uncover critical details during semiconductor quality control with efficiency and reliability using light microscopy
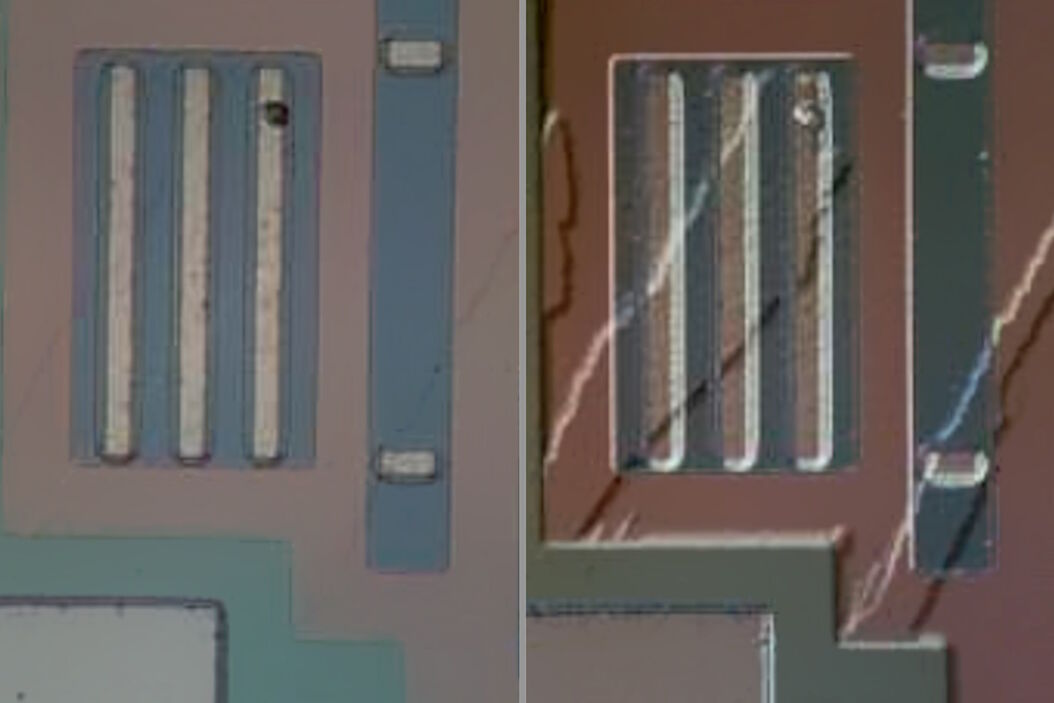
Semiconductor inspection during the production of patterned wafers and ICs (integrated circuits) is important for identifying and minimizing defects. To increase the efficiency of quality control in the early stages of production and to ensure reliable IC chip performance, microscopy solutions should combine different contrast methods that provide complete and accurate information about different defects. Our free guide details microscopy techniques & optimizing quality control. Get your free copy today!
In this article, you will discover
- The importance of early defect detection: Microscopy enables manufacturers to identify potential problems early, ensuring the quality and reliability of the final IC product.
- A spectrum of contrast techniques: Explore the principles behind various contrast techniques, including brightfield, darkfield, polarization, DIC, UV, oblique, and