What is Spatial Biology?
Put simply, spatial biology is the study of the organization and interaction of molecules, cells, and tissues, and the relationships between them, in their native 2D or 3D spatial context. With spatial biology, researchers can explore interactions within native tissue microenvironments, revealing their cellular phenotypic diversity, and spatial architecture.
Spatial Biology Overview articles
How is Microscopy Used in Spatial Biology? A Microscopy Guide
The Shape of the Brain: Spatial Biology of Alzheimer’s Disease
Spatial Architecture of Tumor and Immune Cells in Tumor Tissues
How do I generate spatial biology data?
Spatial biology data is generated using different technology approaches, or often a combination of these approaches, with some of the most prominent including:
- Image-based approaches such as transcriptomics and proteomics using techniques such as antibody-based multiplexing or RNAScope
- Workflows combining mass spectrometry, mass cytometry or sequencing techniques linked to complementary approaches such as laser microdissection which isolates regions, even single cells, for downstream omics analyses whilst preserving spatial context.
AI-based analysis for quantification of spatial relationships is used to complement of all these approaches, and is necessary in order to gain meaningful insights from complex datasets.
Spatial Transcriptomics/Genomics
Uses microscopy, RNA sequencing, in-situ hybridization and other automated counting and profiling approaches to study the transcriptome, or gene expression, with spatial context. For more sensitivity, microdissection techniques are followed by downstream analysis, allowing RNA sequencing reads to be assigned to specific physical locations.
Spatial Proteomics
Uses antibody-based multiplex imaging or mass spectrometry approaches to understand the localization of proteins and their dynamics, with the major differentiator between these techniques being the sensitivity required. Increasingly, imaging is being combined with downstream mass spectrometry, using microdissected tissue regions, with the analyzed data realigned back to the reference images to provide spatial context.
Spatial Metabolomics
Provides quantitative spatial information on non-protein metabolites, such as lipids (lipidomics), sugars (glycomics), and drug molecules, using precise measurement standards to deeply understand human tissue chemistry.
What About Spatial Multiomics?
Spatial multiomics is an emerging area that combines multiple -omics technologies for even greater context and deeper insights. For example, combining proteomics and transcriptomic data with insights into localization within tissue sections.
Laser Microdissection Systems – Preserve spatial context for your downstream proteomics, genomics and transcriptomics analysis.
Laser microdissection in spatial biology research
Deep Visual Proteomics Provides Precise Spatial Proteomic Information
Spatial Biology: Learning the Landscape
The importance of Spatial Biology in Tissue Research
Heterogeneous tissues, such as the tumor microenvironment (TME) are complex assemblies of cells with different phenotypic variations. Investigating the organization and interactions between tumor, stroma and immune cells therefore requires a spatial multiplexed imaging study approach, for which antibodies with high sensitivity and specificity are required. Multiplexed imaging can extract more information from human tissue samples as it allows the visualization of many more biomarkers than traditional microscopy.
Multiplex Imaging of Biomarkers
By observing many biomarkers simultaneously, complex tissue and cell phenotypes can be identified and analyzed. Antibody-based multiplex imaging enables researchers to study when and where proteins are expressed and is used for mapping normal and diseased tissue by cell type, biomarker expression profile, and specific features (known as spatial phenotyping). The spatial mapping and profiling of cells with context provides a deeper understanding of the tissue landscape and disease progression that is crucial for biomarker discovery.
Cell DIVE Multiplex Imaging Solution – Cell DIVE is a precise, open multiplexing solution that lets your research dictate the level of automation required, which antibodies to use, how to build your antibody panel, and more.
What are the different types of Multiplexed Imaging?
Amongst microscopy-based approaches, there are variations in "plexity," , that is the number of analytes analyzed in a sample. Low/mid-plex methods analyze fewer biomarkers, while high-plex methods analyze hundreds or thousands. Researchers may choose lower plex imaging-based techniques, such as conventional light microscopy, for higher resolution imaging of tissue samples. This is crucial when the sample has a complex 3D structure such as spheroids or thick tissue.
Many different methods for multiplexed imaging have become available, and each uses a different approach to achieve higher plexity. The main categories are:
- Single-step staining and imaging, single-round staining (one pass) for a limited number of targets.
- Integrated multi-omics workflows, and iterative staining, enabling detailed analysis of protein distribution and interactions within tissue samples.
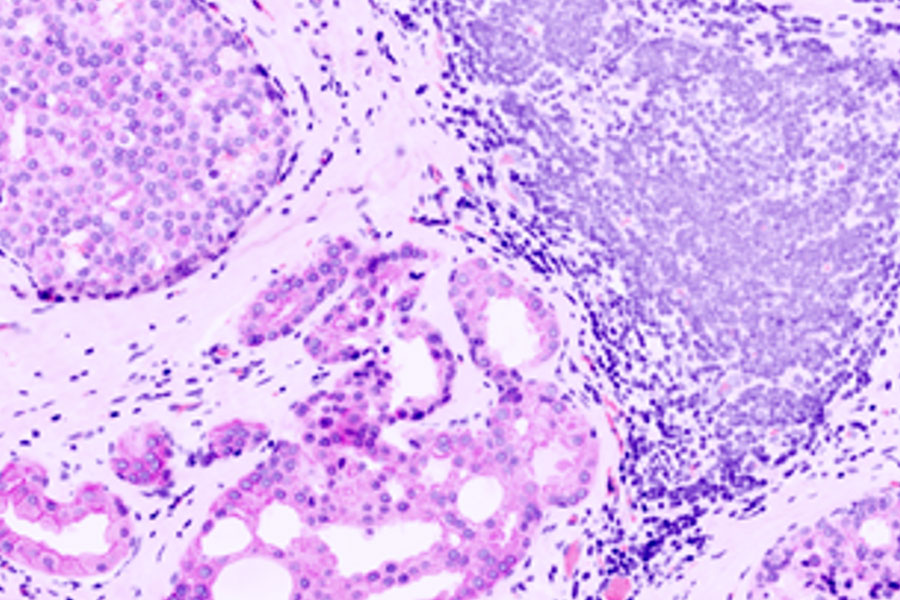
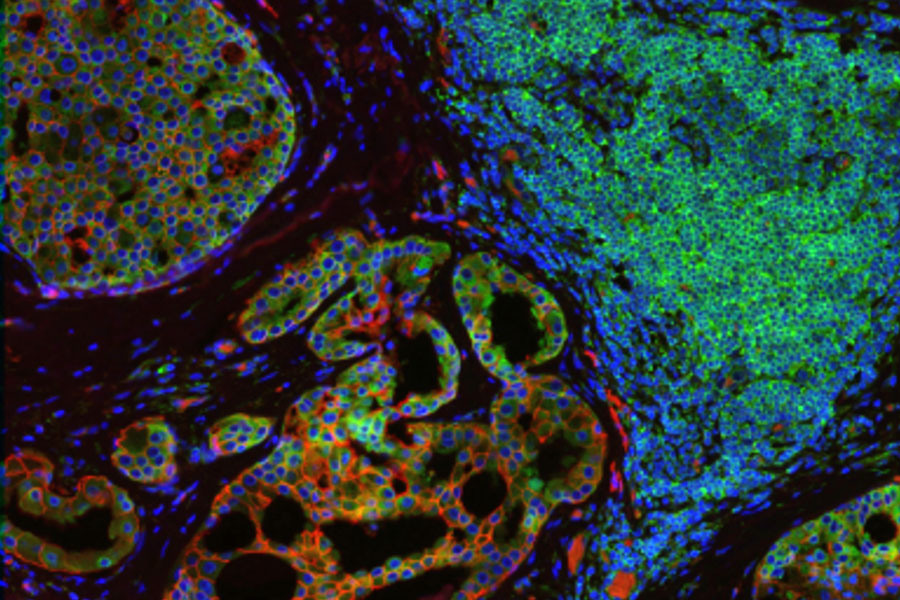
Multiplex Imaging in spatial biology research
Multiplexing with Luke Gammon: Advance your Spatial Biology Research
Empowering Spatial Biology with Open Multiplexing and Cell DIVE
Methods to Improve Reproducibility in Spatial Biology Research
AI-Powered Multiplexed Image Analysis to Explore Colon Adenocarcinoma
IBEX, Cell DIVE, and RNA-Seq: A Multi-omics Approach to Follicular Lymphoma
Accelerating Discovery for Multiplexed Imaging of Diverse Tissues
Dissection of target regions for focused Spatial Biology Analysis
Specific areas of tissue samples, or even individual cells, often need to be isolated either for downstream analysis with high sensitivity, e.g. omics-based approaches, or for further investigation of a specific section without contamination from surrounding cells. For example, in cancer biology, there are distinct molecular differences between tumor and non-tumor regions, as well as within the tumor itself. These differences can only be deciphered by isolating specific sections of these regions. Laser microdissection (LMD), also known as laser capture microdissection (LCM), is increasingly being used in spatial biology to isolate and dissect single target cells or entire areas of tissue from a wide variety of samples. This can be coupled with artificial-intelligence (AI)-guided approaches to automatically define regions of interest (ROI) which are destined for dissection.
An Introduction to Laser Microdissection – Precise location or separation of single cells and tissue structures
The emergence of AI in Spatial Biology
Spatial biology technologies can generate vast amounts of data, often in the form of images, holding within them the answers to many research questions. However, the vast volume and complexity of this data makes analysis challenging. Furthermore, when analyzing multiple labels and thousands of data points, overcoming the subjectivity of analysis can be a significant hurdle. These factors are helping fuel a growing trend in the use of AI-powered multiplexed image analysis to derive meaningful and quantifiable insights from spatial data. For example, AI-driven machine learning analysis of heterogeneous tissue samples can reveal unique and previously unidentified cell phenotypes and their distribution within the tissue microenvironment. Such insights can enable scientists to better categorize tumor types and make more accurate predictions of responses to therapy, ultimately leading to improved patient outcomes.
AI-based analysis in spatial biology research
Dive into Pancreatic Cancer Research with Big Data
Explore Alzheimer's Spatial Proteome with Big Data
Uncover the Hidden Complexity of Colon Cancer with Big Data
AI-Powered Multiplexed Image Analysis to Explore Colon Adenocarcinoma
3D Spatial Analysis Using Mica's AI-Enabled Microscopy Software
Conclusion
Spatial biology encompasses tools, methods, and analyses that combine different technologies such as -omics technologies with imaging-based approaches to gain positional information, enhancing our understanding of tissue organization and spatial interactions. As these technologies advance, the range of questions researchers can address will become more sophisticated. Complex areas like cancer biology, neuroscience and developmental biology are poised for significant impact from these breakthroughs. Adopting the spatial biology mindset will facilitate the integration of these methods and inspire researchers to explore new approaches in their experimental systems.
Online learning resources
See the Hidden: Spatial Biology I
Discover new approaches that leverage fluorescence multiplexing and spatial omics to examine the behavior and identity of cells in diverse tissues, as well as identifying local changes in protein content.
VIEW ON DEMAND
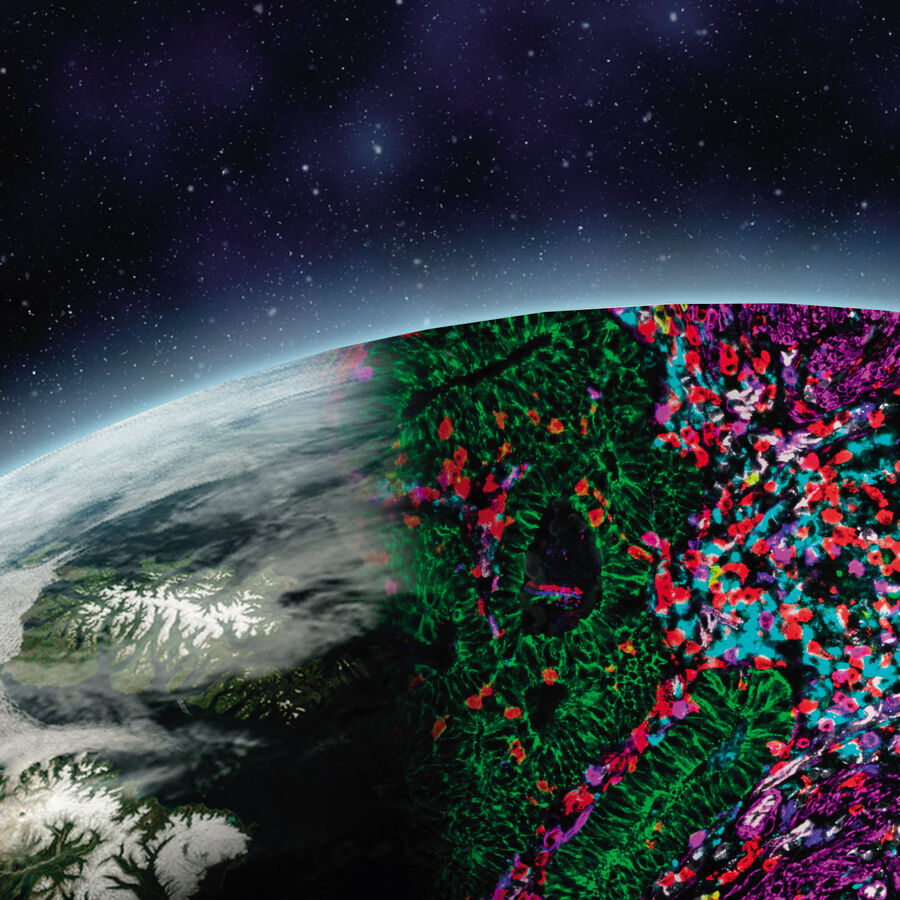
See the Hidden: Spatial Biology II
Examine the spatial mapping of single cells within context, focusing on the tissue microenvironment, as well as techniques to analyze variability in spatial single-cell RNA and protein expression.
See the Hidden: Spatial Proteomics
Learn how a deeper understanding of protein subcellular localization, using advanced microscopy techniques, can shed new insights into diseases such as metabolic disorders and cancer.
VIEW ON DEMAND
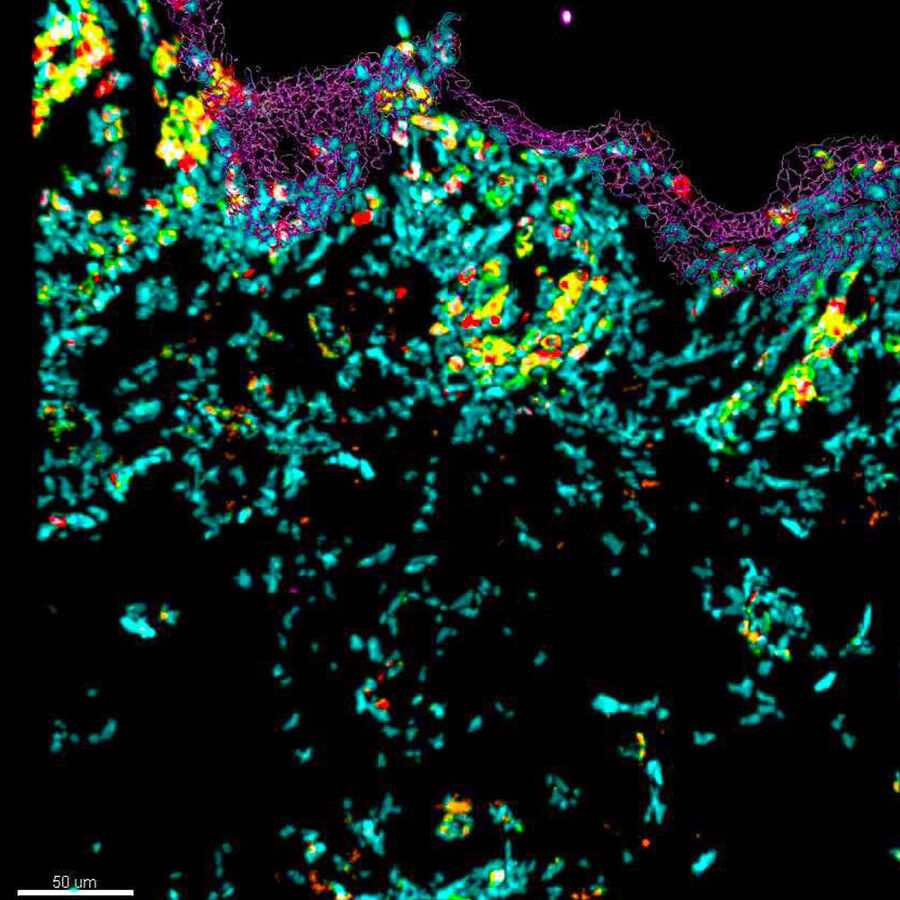
See the Hidden: Spatial Proteomics II
See how 10-color image acquisition using a confocal microscope masters the challenge of identifying skin immune cells in healthy and pathological samples.
VIEW ON DEMAND
Spatial Biology FAQ
Spatial biology combines spatial positional data with molecular information, allowing researchers to study the organization and function of biomarkers within their native tissue environments. This approach assigns spatial coordinates to identified biomarkers, creating detailed maps of their distribution and interactions.
Spatial biology techniques provide high-resolution insights into the spatial organization of different cell types in the tissue which can be precisely phenotyped using multiple biomarkers. This is crucial for understanding complex biological processes, such as tumor microenvironments, immune cell interactions, and developmental biology. By revealing how cells are arranged and interact in situ, spatial biology can inform therapeutic strategies and improve predictions of treatment responses.
Spatial biology helps researchers understand the organization of cells and biomarkers within tumor microenvironments. Key questions, such as the proximity of tumor cells to blood vessels, the presence and state of immune cells, and tumor heterogeneity in gene and protein expression, can be addressed using spatial biology techniques. These insights are vital for predicting responses to immunotherapies and other treatments.
Combining antibody-based multiplexed imaging with specific cell-type markers, can provide a better understanding of the spatial heterogeneity of the brain and its contribution to neurodegenerative diseases. These insights can ultimately lead to more effective therapies for the treatment and prevention of conditions such as such as Alzheimer’s disease.
Spatial biology utilizes a combination of advanced technologies, including:
Sequencing: Spatial transcriptomics and spatial genomics assign RNA and DNA sequencing reads to specific tissue locations.
Mass Spectrometry: Spatial proteomics and spatial metabolomics analyze proteins and metabolites within tissue regions.
Fluorescence Imaging: Visualization and quantification of low plex markers in 2D and 3D samples using conventional microscopy, providing high-resolution spatial data, or analysis of tens to hundreds of proteins in a sample using antibody-based multiplexed imaging.
Laser Microdissection: Allows isolation of tissue samples, even single cells, for downstream spatial omics analysis, or for investigation of specific regions, free of contamination.
AI-based image analysis: Exploring and visualizing complex data and spatial relationships in 2D and 3D multiplexed images.
Spatial biology is ideal for studying complex, developing tissues, such as hair follicles, which display granular cellular identities that change with the tissue’s lifecycle. It helps researchers understand how stem cells move through different niches and provides granular knowledge of cellular identity and position, essential for developmental biology research.
Whilst both of these approaches use fluorescence microscopy, low- to mid-plex techniques analyze fewer biomarkers and offer higher resolution, making them suitable for detailed studies of specific analytes within single cells. High-plex techniques analyze hundreds or thousands of biomarkers, providing comprehensive insights into complex tissues. Researchers often start with low-plex techniques to define hypotheses and examine specific tissue regions, followed by high-plex methods for more extensive analysis.
Multiplexed imaging in spatial biology involves various workflows that use different technologies, such as:
Staining and Imaging in One: Combines staining and imaging in a single step.
One-Pass Lower-Plex: Uses a single round of staining to analyze a limited number of targets.
All-in-One Omics Solutions: Integrates multiple omics techniques into a single workflow.
Iterative Staining: Involves multiple rounds of staining and imaging to build a comprehensive profile of the sample. This approach provides detailed spatial information about protein distribution and interactions within tissues.
Cell DIVE-IBEX is a hybrid imaging method that combines the strengths of Cell DIVE and IBEX (Iterative Bleaching Extends Multiplexity) to provide comprehensive multiplex imaging. The IBEX method was invented by the laboratory of Ronald Germain at the National Institutes of Health in Bethesda, Maryland. It integrates the antigen retrieval, antibody staining, and dye inactivation techniques of IBEX with the advanced imaging and software capabilities of Cell DIVE. Cell DIVE-IBEX enables detailed and comprehensive analysis of tissue samples, providing a deeper understanding of the spatial relationships and interactions between different cell types and biomarkers. However, image analysis of multiplexed datasets remains a challenge. Fortunately, artificial intelligence (AI)- based image analysis has advanced significantly through innovations in deep learning, accurate segmentation, and automated phenotyping, making it a powerful tool for researchers. By combining the Cell DIVE-IBEX method with AI-powered image analysis, researchers can study cellular interactions and heterogeneity within the tumor microenvironment, significantly improving the accuracy, efficiency, and depth of spatial biological insights.