Content
This application note describes:
- For organic-rich shale rock:
- measurement of pore geometries of the physical pore space in the representative elementary area (REA) and assessment of pore-mineral associations; and
- correction of bulk porosity and permeability measurements for the influence of core damage, like cracks, to attain more accurate results;
- For carbonate rock (travertines):
- analysis of pore size distribution and total porosity
Introduction
Organic-matter porosity is an important property of mature organic-rich shales providing storage capacity for liquids and gases [1]. Wettability, in the sense of fluid distribution, saturation, and flow in porous media, is a crucial petrophysical property of reservoir carbonate rock [2]. It is affected by the distribution of gas, oil, and water within reservoir rocks and the capillary pressure and relative permeability.
Measurements of porosity in shale and carbonate rocks at high resolution are difficult to do in a representative way. The difficulty arises from:
- heterogeneity of samples and small pore sizes < 5 μm in shale [3] and
- large variation of pore size in carbonate rocks [4].
Using ViP (virtual petrography) and BIB-SEM (broad ion beam milling and scanning electron microscopy), the porosity was investigated:
- in shales to measure pore geometries in the REA (representative elementary area) and mineral composition of pore areas (BIB-SEM) [3] and
- in carbonates at the macroscale (ViP) and microscale (BIB-SEM) to determine the range of pore sizes [4].
BIB-SEM enables representative assessment of microporosity in both shales and carbonates with nanoscale resolution. Moreover, with BIB-SEM, the core damage in shales, like cracks, can be quantified and its influence removed from the bulk porosity and permeability measurements. With the combined use of ViP and BIB-SEM to characterize carbonate rocks, the porosity, pore size distribution, pore geometry, and pore type classification can be determined at multiple scales.
Material and methods
VIP
Virtual Petography (ViP) is an automated optical microscopy imaging technique, developed by the Fraunhofer Institute for Applied Information Technology (FIT) in Germany, exploiting transmitted, polarized, and reflected light for sample illumination. It acquires mosaic images of petrographic samples with 10 nm accuracy and creates a highly compressed gigapixel image map. Fracture networks, minerals, pores, and mineral overgrowths in rocks can be segmented using multiscale, automated petrographic analysis. With the ViP method, several hundred thousand pores can be segmented accurately within a short time based on the extinction behavior of each pixel while recording with cross-polarized light and interpolating from several rotation angles. Regions of interest on a sample can be selected for enhanced analysis with methods like BIB-SEM.
BIB-SEM
Broad ion beam (BIB) milling of shale and carbonate samples, for the data reported here, was done with a JEOL BIB cross section polisher. Another BIB milling solution is the EM TIC 3X system from Leica Microsystems, which was used for additional data reported in reference [1]. Before the BIB-milled samples were placed in the SEM (Supra 55, Zeiss), they were sputter coated with a thin layer of tungsten (W) using the EM ACE600 sputter, carbon, and e-beam coater from Leica Microsystems. The W layer avoids charging of the non- conductive samples when irradiated by the electron beam.
Shale samples
Samples of Posidonia shale rock were used. The shale has a total organic carbon (TOC) of 8% (wt), mostly kerogen type II [3]. The composition is mainly carbonate, fossil, quartz, feldspars, clay mineral, and framboidal and euhedral pyrite grains.
Shale samples were BIB-milled, producing a damage free, flat cross section of 1.5 mm² area, before imaging with the SEM. The REA was determined by box counting in the backscattered electron (BSE) image, which was segmented into 4 phases, namely: 1) organic matter, 2) clayey matrix (including quartz and feldspars), 3) carbonates, and 4) pyrite along with other relatively dense minerals. A large carbonate shell found in the shale cross section was excluded from analysis (Figure 1). High resolution, gigapixel mapping of the pore space was performed on the area of interest with a pixel size of 15 nm using the secondary electron (SE) detector. The pore space was segmented from the SE map by applying advanced BIB-SEM image processing algorithms. Composition of matter within or around a pore was determined with energy dispersive x-ray spectroscopy (EDS). Mineral related porosity was determined by combining the segmented pore space with high resolution BSE (30 nm pixel resolution) and EDS (60 nm pixel resolution) maps of the shale.
Carbonate samples
Samples of limestone (quaternary continental carbonate rock or travertine) from the Lixhe region in Belgium or the Maastricht area in the Netherlands were used [4].
Characterization of the macroporosity of the carbonate samples was carried out with ViP which generated maps of the sample using polarized light collected at different polarization angles. The sample porosity is segmented in the gigapixel map data based on the non-existing extinction behavior of the pore space.
Microporosity characterization of the carbonate samples was performed with BIB-SEM. The pore space was segmented from the ViP and BIB-SEM image data using advanced image processing algorithms.
Results
Shale
REA and Porosity
The box counting in the BSE image map shows the shale REA is approximately 100 x 100 μm2 for this BIB cross section (Figure 2). Segmented organic matter is 18 ± 1% (vol), which corresponds to the average TOC of 8% (wt) [3]. The composition of the box has relatively more organic matter and clayey matrix and relatively less carbonate and pyrite compared to the average composition over the entire area of interest (Figure 3). However, the differences are generally < 5% (vol).
The visible porosity in the segmented BSE map (Figure 4) is 2.6% and contains over 20,000 pores and about 750 cracks [3]. These cracks account for about 1/3 of the visible pore space. The pores are mainly < 1 μm in equivalent diameter and follow a power-law distribution indicating a fractal geometry. This fractal geometry allows extrapolation of the power-law distribution to smaller pore sizes and comparison to mercury (Hg) intrusion porosimetry (MIP) data. MIP allows pore size to be determined based on the external pressure needed to cause the intrusion of Hg into a material’s pores. The comparison shows that pore and crack porosity values extrapolated from BIB-SEM data are comparable to those from MIP data (Figure 5). This result indicates that MIP does not detect the cracks revealed by SEM imaging (Figure 4). Moreover, extrapolation of the pore image data leads to higher porosity values compared to MIP, which is due to the isolated intra-particle pores.
Combining the segmented pore space with the BSE and EDS maps reveals that only a small fraction of the pores is located within the organic matter (Figure 6) [3]. Most of the pores are present in the carbonate grains and fossils. The other part of the pore space is made up of inter-particle pores between the clay minerals, quartz, feldspars, and carbonate grains.
Carbonate
ViP Macroscale Porosity
ViP image data of the carbonate sample recorded with plane or linear polarized light (PPL) and crossed polarized light (XPL) are shown in Figure 7. The porosity is corrected for organic matter which is segmented from the map using information from PPL, XPL, and interpolated XPOL image data (example in Figure 8) [4].
BIB-SEM Microscale Porosity
The BIB milled surface allows gigapixel image mapping of the microstructure with nanoscale resolution. Image processing enables segmentation of the microporosity (Figure 9) [4].
Pore Size Analysis
Over 100,000 pores were segmented from the thin section and more than 17,000 pores from the BIB-SEM image map. A plot of the number of pores, normalized by the sample area, versus the pore area in nm2 on a log-log graph shows a linear trend (Figure 10) [4]. This trend indicates a fractal distribution of the pore sizes. Moreover, the lines of best fit show essentially a perfect linear correlation with the data (square of correlation coefficient, R2 = 1), indicating how representative the data really are. When both the microporosity data acquired by BIB-SEM and the macroporosity data acquired by ViP are cumulated and plotted (Figure 11), the porosity is 19%. Extrapolating the cumulative porosity, based on fractal distribution data, down to nanoscale pore sizes results in an upper porosity value of 20%.
Summary and conclusions
For fine-grained shale rock, broad ion beam milling and scanning electron microscopy (BIB-SEM) can be used to analyze the microstructure and porosity in a representative elementary area (REA), while still correcting for core damage, such as cracks [3]. Quantification of the visible pore sizes can be compared to bulk porosity measurements, like mercury intrusion porosimetry (MIP). Combining different types of image data enables advanced analysis, such as mineral-pore associations.
For travertine carbonate rock, microscale and macroscale porosity analysis is achieved by exploiting both virtual petrography (ViP) and BIB-SEM image mapping technologies [4]. Combined analysis of both data sets delivers representative porosity results.
Download the Application Note as PDF
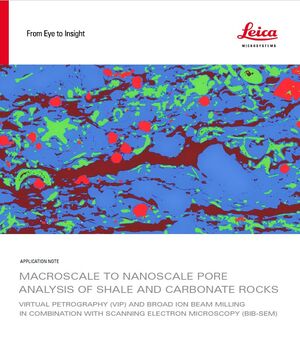
Click here to download the application note as pdf.