Cryo Electron Tomography workflow and why a cryo light microscope is essential
Many scientific questions concerning the physiology and pathology of the human organism can only be answered by investigating the underlying cellular mechanisms. They are the foundation of any functional tissue, organ and the whole organism.
For understanding the function of different cell types under healthy and pathophysiological conditions, it is crucial to determine the participating biomolecules, such as proteins, and to examine their interaction at the molecular level.
To achieve this, Cryo Transmission Electron Microscopy (Cryo TEM) is used to resolve biomolecules within their cellular environment down to an unprecedented resolution below 1 nm. In this way individual proteins can be recognized without any labeling, “just” by their shape. Furthermore, even different conformations and their distribution within the cytoplasm can be distinguished.
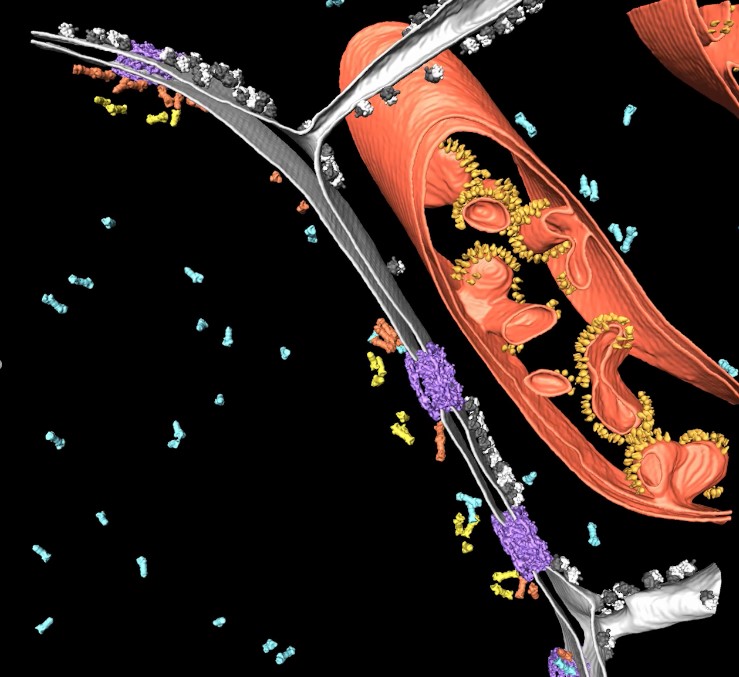
As a precondition, specimens have to be cryo-fixed by using sophisticated techniques (vitrification) avoiding destructive ice crystal formation. In contrast to other fixation techniques the proteins are maintained as close to the native state as possible. Afterwards, thin specimen (less than 300nm) can be directly assessed in the Cryo TEM. By tilting the sample, a 3-dimensional dataset of the observed sample volume can be created and reconstructed to obtain a 3D distribution of the relevant proteins of interest (Figure 2, Cryo Electron Tomography).
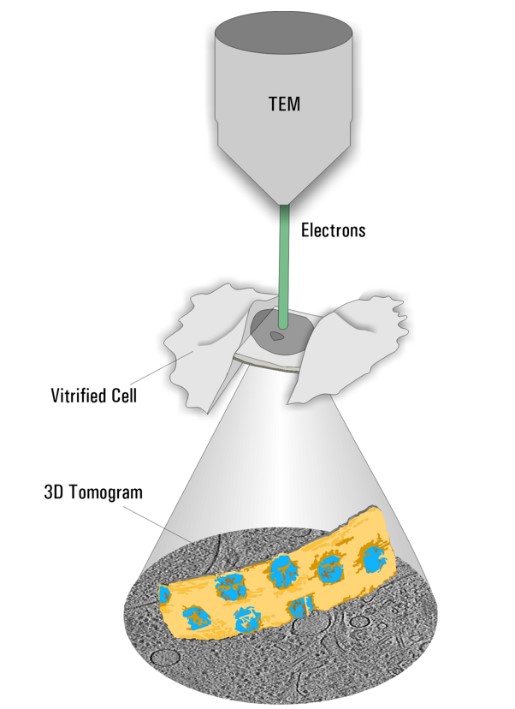
For observation of “thicker” parts of the specimens, the sample must be thinned. Beside cryo ultramicrotomy, Focused-Ion-Beam (FIB) Milling using a dedicated Cryo Scanning Electron Microscope is the method of choice. Two ion beam windows are positioned in a way that a thin ice sheet (lamella) of about 200 nm thickness is created at the area of interest. Like this, even parts of specimens, which could not be investigated are accessible for Cryo ET (Figure 3, FIB Milling).
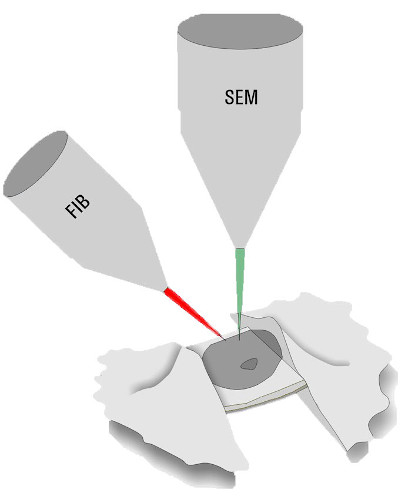
As the workflow is tedious, many steps are needed while material and imaging time on the EMs is expensive, it is crucial to determine the quality of the sample and the presence of the target on the grid at an early stage. Furthermore, one major challenge is to find the precise milling positions to ensure that the lamella contains the protein(s) of interest.
To overcome these challenges, a cryo light microscope is an essential component of the workflow. Using a cryo light microscope the quality of the samples can be checked and, most importantly, the position of a structure of interest can be determined by using genetically encoded fluorescence tags (Figure 4).
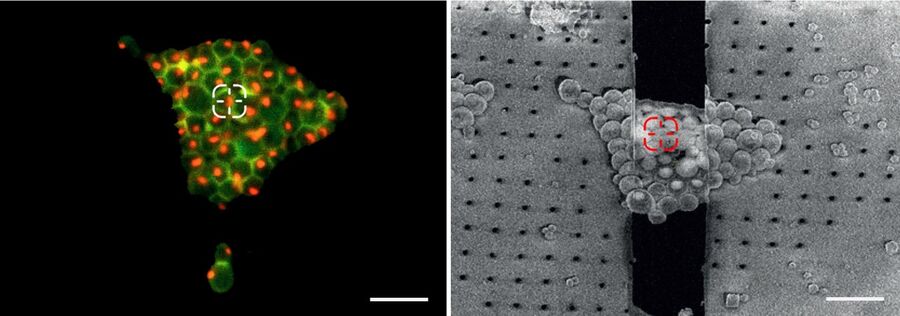
These markers can be visualized selectively and reveal the position of any structure of interest in 2D and 3D. Correlating the fluorescence and SEM images allows potential milling sites to be allocated (Correlative Light and Electron Microscopy, CLEM).
Although many challenges appear (safe cryo transfer of samples, potential water condensation on cold parts, objective used at low temperatures…) commercial cryo light microscopes are available.
Cryo light microscope and haze removal
Leica Microsystems provides a dedicated cryo light microscope, the THUNDER Imager EM Cryo CLEM, which is equipped with latest LED-technology and a highly sensitive, state-of-the-art scientific